The Latest From the Labs
University researchers around the country are leading us back to the future
Imagine a country where clean, green electric vehicles dominate the roads and rails, offering drivers and passengers the greatest range, performance, reliability, and price. Where electricity, not oil, is king. And where gasoline is little more than a niche product.
Welcome to the United States of America, circa 1900.
The primary use for gasoline a century ago was as a remedy for killing head lice. The cars that could go the farthest and fastest were electric. Electric trolleys and trains were ubiquitous, handling 12 billion passenger trips a year. Even after Henry Ford started building internal-combustion-powered cars for the masses, he bought quiet, safe, clean electric models for his wife and son. And he plotted with Thomas Edison to design electric cars that could go 100 miles or more on a single charge. The possibility of an energy and transportation future in which peak oil, climate change, and devastating oil spills were not looming calamities was perfectly plausible.
Of course, the United States and other countries took a very different road. And not because fossil fuel technology is inherently better—in fact, the 2008 Ford Explorer gets fewer miles per gallon than the 1908 Ford Model T did. And even if it used electricity generated by burning coal, electric transportation would emit 40 percent less carbon than is spewed by internal combustion.
The main reason for the move to petroleum was the nation's then-primitive infrastructure. There was no utility grid as we know it. Most homes didn't have electricity, and where it was available, it was far more expensive than it is today and virtually impossible to store. Fossil fuels were plentiful, portable, and exceedingly cheap—if you ignored their environmental impact, which we blithely did.
Now research scientists are trying to turn back the clock. They are working on a method of energy production that won't pump out greenhouse gases but rather will remove them from the air; superefficient batteries and nanotechnology that will drive the next generation of vehicles and consumer devices while bolstering the electric grid with stored energy; carbon-neutral biofuels to replace carbon-spewing fossil fuels; and a zero-carbon solar energy system that will electrify the planet 24-7.
Here's a look at six innovators whose projects could take us well beyond a future that was once within our grasp.
On the Moove
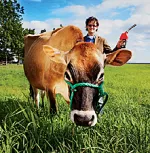
Professor Eric Leonhardt and his students at Western Washington University's Vehicle Research Institute have built a hybrid car that gets the equivalent of 94 miles a gallon while using a fuel made from a plentiful local waste product: cow manure. The most revolutionary aspect of this research isn't the source of the fuel or the mpg, however. It's the biofuel's projected equivalent cost per gallon: about $2.50.
Leonhardt says he and his team have found a way to make manure biogas that's both suitable for vehicles and competitive in price with fossil fuels. The university just received a half-million-dollar Department of Energy grant to expand the project. To perfect the technology, Leonhardt's team built a mini-refinery at the fuel source—Vander Haak Dairy, near the university's Bellingham campus. The device scrubs the biogas that comes off decomposing manure, turning a noxious mix of gases that contains 60 percent methane into pure biomethane.
"It's a huge net winner for the environment," Leonhardt says. "Not only are we removing a major source of methane emissions, which are 23 times as potent as carbon dioxide as a greenhouse gas, but we're also displacing fossil fuels by using the biogas in hybrid vehicles."
His team can produce the equivalent of 100,000 gallons of gasoline per year from the manure at the small dairy, which has just 667 cows. If all 80,000 dairy cows in Washington's Whatcom County were tapped for biogas, he says, they could produce the equivalent of 13 to 20 million gallons of gasoline and offset the CO2 emissions of 130,000 cars.
Nationwide, there are 9 million dairy cows. Putting all their manure to work through Leonhardt's process could yield the equivalent of more than 2 billion gallons of gas and carbon offsets equal to the emissions of more than 3 million cars.
The Washington biogas project got its start in 2004, when Vander Haak Dairy installed a methane digester—basically a covered concrete box in which manure decomposes and produces biogas. A converted diesel generator burned the raw gas and produced electricity, but the system, though cost-effective, couldn't compete with the state's low-cost hydropower, and much of the biogas ended up getting flared before it could be used to power the farm or the grid.
Using the gas to make biomethane for vehicles or power generators turned out to be a more viable solution. "The so-called experts at the EPA told us over and over that it could never be cost-effective," Leonhardt says. "Maybe they have bad data, because when we tried it, there was no significant cost. It's a great solution."
Silt Solution
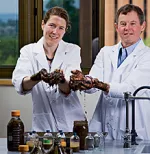
It seems fitting that the environmental Havoc wrought by humans could be resolved in large measure by a microorganism. At least Derek Lovley thinks so. The University of Massachusetts Amherst microbiology professor is experimenting with bacteria he discovered in the slimy silt of the Potomac River. The anaerobic organisms inhale carbon dioxide, feed on electricity, then produce biofuels that pack an energy punch similar to that of gasoline.
"The beauty is that you can do it anywhere—at power plants, on rooftops," Lovley says. "You don't need huge amounts of arable land, water, and fossil fuel inputs, as with other biofuels."
If the electricity fed to these microscopic refineries is renewable—like that produced by the solar panel in Lovley's lab—then the resulting biofuel can't worsen the climate crisis. It is, as he says, a carbon-neutral closed loop: Burning the fuel in cars or power plants would only release the greenhouse gases that the bacteria had previously absorbed.
The electricity-eating bacteria's behavior mimics light-dependent photosynthesis in plants so closely that Lovley and other researchers have taken to calling it electrosynthesis. But the bacterial process is about 1,000 times more efficient. Lovley believes that electrosynthesis could produce car-worthy butanol for two bucks a gallon. And it could also solve a major hurdle for renewable energy: intermittency. By storing energy generated from solar cells and wind farms in the form of microbial biofuels, renewable-energy power generators could run around the clock.
Lovley discovered the so-called Geobacter organism in 1987 while investigating what was happening to the metals and phosphates from detergents and fertilizers in the Potomac's sediment. He found that Geobacter rendered harmless a variety of nasty pollutants. (He's currently using them to protect groundwater in Colorado from uranium contamination.) In experiments, the useful organism also produced electricity under certain conditions, so Lovley and others began working on a microbial fuel cell.
The biggest breakthrough came when Lovley figured out how to feed electricity and carbon dioxide to the critters, which love living on the surface of electrodes, where they spit out complex organic molecules—including biofuels. His most recent experiments have used a cousin of Geobacter called Sporomusa ovata, whose genome could be tweaked to make butanol—which doesn't mix with water and has about 95 percent of the energy density of gasoline, meaning that almost any engine that uses gasoline would work just fine on microbial butanol.
The U.S. Department of Energy just sent UMass a million dollars to help Lovley's lab scale up this technology. In fact, the DOE was so impressed with the potential that it has so far doled out more than $41 million to 13 projects to accelerate the development of Lovley's new class of green electrofuels.
Climate Scrubbers
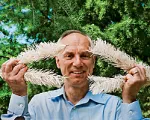
A geophysicist by training, Klaus Lackner has hunted quarks with Nobelist Martin Perl and investigated nuclear fusion at Los Alamos National Laboratory. But it was his daughter's eighth-grade science fair experiment that set him on his current and most urgent quest: countering climate change by inventing a practical, affordable method for capturing carbon dioxide from the air. His solution: a synthetic tree that absorbs a thousand times more carbon dioxide than the real thing.
"In the lab it looks more like a pine branch than a whole tree," Lackner, director of the Lenfest Center for Sustainable Energy at Columbia University, says of the carbon-dioxide-absorbing resin he developed and shaped to resemble a branch of pine needles. "We call it the 'bottle brush.' On a commercial scale, it would be a whole tree made of these branches."
Lackner's path was set 11 years ago when his daughter Claire used an aquarium pump and lye (sodium hydroxide) to capture about half the carbon dioxide contained in a test tube filled with air. Lye, a corrosively strong base, soaked up the acidic carbon dioxide with far greater ease than he'd expected.
Inspired, he began testing substances less harmful than lye and eventually developed a carbon-collecting resin that can be shaped into spongelike branches. The resin has the added property of absorbing carbon dioxide when dry and releasing it when wet—making what could be a costly, energy-intensive process of scrubbing carbon into a relatively simple, inexpensive task. The synthetic branches are designed to rotate hourly into a vacuum chamber, where they are soaked in water and release the carbon dioxide, which is pumped away by a compressor.
Each "bottle-brush tree" would extract a ton of carbon dioxide from the air each day, but even at that rate, reducing annual global carbon emissions by 10 percent would require "planting" more than 8 million of the devices. Lackner says that's not such a big number, noting that more than 70 million cars and light trucks are manufactured each year; he figures that each of his trees would cost no more than the average car (compared with the price of about $2 million for a single commercial wind turbine).
While Lackner believes renewable power, particularly solar, must provide the long-term solution for the world's energy needs, he argues that action must be taken now, in the short term, while we still live off fossil fuels. He took this message to Congress earlier this year when he testified that carbon capture provides the best available option for stabilizing the climate while we work toward a renewable-energy future.
If Congress puts a price on carbon, through either cap and trade or a carbon tax, the demand for his synthetic trees could be huge. Even more alluring, Lackner says, is the possibility of selling the compressed CO2 gathered by the trees to make synthetic fuels or biofuels—as with the bacterial fuel factories being developed at Derek Lovley's University of Massachusetts lab (see opposite page).
Batteries That Pack a Punch
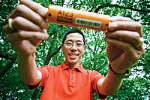
When the Formula One racing association sought hybrid technology to give its race cars a speed boost, Massachusetts Institute of Technology professor Yet-Ming Chiang and his colleagues saw a chance to showcase the revolutionary lithium-battery chemistry he was developing for a new generation of electric and hybrid vehicles.
So he and his colleagues came up with a battery that's just a bit bigger than a double-A alkaline but can pump out one full horsepower of energy, several orders of magnitude greater than the typical AA. Then he made power packs holding 80 of these firecracker batteries for vehicles on the McLaren Formula One team, which gave eight-second, 80-horsepower boosts that allowed racers to roar past competitors with astonishing bursts of acceleration. The costly technology provided such an impressive advantage that the racing association put a moratorium on the power packs until every team could afford them. "Let's just say that the drivers using our system passed other cars," Chiang recalls. "A lot of cars."
The Formula One experiments showed off one of the defining qualities of Chiang's batteries. They are very good at using up most of their stored energy, then charging very, very fast.
While other battery chemistries boast more energy or more storage than Chiang's, they can only safely discharge a portion of their energy, which means their usable energy isn't even close to their theoretical capacity. Chiang solved this limitation by abandoning conventional cobalt-based cathodes used in other lithium batteries in favor of the nanoscale phosphates he developed in his MIT lab. The new chemistry lets his batteries achieve 5 to 10 times the discharge rates of the cobalt systems, he says, resulting in batteries that can pack more usable power into a smaller package.
Chiang licensed his invention from MIT and in 2002 formed A123 Systems to refine the technology and bring it to market. The company's first product was a battery pack for DeWalt power tools, which made the drills, saws, and other cordless equipment more powerful than those of competing brands. "That got people's attention," he says.
Next, A123 Systems built a larger-format car battery using Chiang's phosphate chemistry and installed it in his Toyota Prius, allowing it to get as much as 120 miles a gallon. Several carmakers have ordered A123 batteries. Fisker Automotive plans to bring a new hybrid to market next year that will go a minimum of 50 miles before burning a drop of gas.
Chiang is trying to develop a next-gen battery with a $5 million DOE advanced research grant. The U.S. government gave A123 $249 million in green stimulus funds to convert an abandoned factory in Michigan that once manufactured Disney videos.
A few years ago, Chiang says, government and industry overlooked battery research in favor of eye-popping (and ultimately failed) claims that a new hydrogen economy was just around the corner. "When we started in 2002, it was a huge risk, because no one seemed interested," he says. "Now the industry is seeing an incredible rate of progress."
A little Gold, a Lot of Power
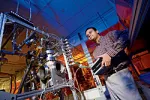
In a breakthrough that could someday spell the end of batteries in consumer electronics, scientists in Maryland and Pennsylvania have created the first circuits that can power themselves with light.
This new optical-circuit technology is distinct from photovoltaic solar cells that generate electricity from sunshine, which is then directed into electrical circuits. The new self-powering circuits can use light from any source—natural or artificial, and any color of the light spectrum.
"You could make a desktop computer in a glass case, and room light could drive all the circuits on the motherboard," says Parag Banerjee, a materials scientist working on his Ph.D. at the University of Maryland. "It would need no input of electricity to run." Cell phones, iPods, and other handheld devices could be engineered to work without batteries. That could put a stop to "vampire electronics"—rechargers, AC adapters, and instant-on power sources. Five percent of U.S. residential electricity use is devoted to such electricity vampires, which suck power whether or not a device is in use.
Banerjee's optical circuit, developed while he was working with Professor Dawn A. Bonnell in her University of Pennsylvania materials research lab, is constructed out of gold nanoparticles—tiny bits that have the ability to give up free electrons. The gold is joined microscopically to a synthetic organic molecule called a porphyrin, triggering a process similar to photosynthesis in plants. Once joined, the particles create an electric current when exposed to light, thanks to a phenomenon known as "surface plasmons." The unique, light-sensitive properties of plasmons have been exploited for centuries; medieval stained-glass artisans used them (albeit unwittingly) when they added bits of gold to make vibrantly red glass.
Other possible applications for the technology include touch screens that serve as both power source and computer interface, miniature robots powered by light, and computer processors and hard drives that forsake conventional logic circuits for systems that use a rainbow of wavelengths of light for unheard-of speed and power.
The discovery was a coup for Banerjee, who had worked for years in the private sector on capacitor technology before he entered the University of Maryland's Laboratory for Advanced Materials Processing in search of a new challenge. He is now using his nanoparticle expertise to build a better solar power cell for larger-scale power generation. Instead of employing variations of the silicon in today's most powerful solar panels, he wants to engineer a system built out of inexpensive and plentiful zinc oxide—best known as sunblock.
"We're only beginning to explore the possibilities of these new materials and technologies," Banerjee says. "The question is scalability. But we will get there."
New Life for Batteries
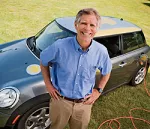
Many Researchers have joined what is now a global effort to create a new generation of hybrid and electric vehicles (EVs) capable of supplanting those with internal combustion engines. But the University of California at Davis has one of the few research centers in the nation, if not the world, where a different yet no-less-critical question is being considered: What happens after hybrids and EVs win?
Sooner or later, the cost and scarcity of oil, fossil fuel's environmental consequences, and the painless advantages of hybrids will combine to undo internal combustion's dominance, says Tom Turrentine, director of UC Davis's Plug-in Hybrid Electric Vehicle Research Center. "We are trying to prepare for that day."
What, for instance, happens to the grid when 100 million plug-in cars pull into their garages? And what are we going to do with all those batteries once they reach the end of their life span?
"The last thing we want to see is a flood of batteries heading to landfills," Turrentine says. "Instead, we're trying to look at them as a resource."
The thing about electric vehicles' batteries is that they are built to perform under stress—high charge and discharge rates, temperature extremes, bumpy roads, lead-footed drivers. After 10 years, most batteries decline in performance. But even though they might no longer be suitable for vehicles, they could still have decades of use if they were clustered as stationary storage batteries in the relatively undemanding environment of a garage.
Such battery packs could provide a means of distributing and storing electric power either for home use or to supplement grid power during peak times. A smart (i.e., computerized) grid would know when to tap that stored energy and when to charge those batteries. The battery packs could also store energy gathered by solar panels and home windmills during the day, then move it into a garaged electric car at night.
Under a $3 million contract with the California Energy Commission, the Davis center is studying the "second life" of advanced car batteries. Researchers are looking at the feasibility of converting used plug-in hybrid- and electric-car batteries into garage installations that would become part of the smart grid planned in California and nationwide.
"In California, we need storage, particularly for renewables," Turrentine says. "Repurposing car batteries is one possible solution." The center is overseeing a three-year test using lithium-ion batteries. Preliminary results are due early next year. Turrentine, an anthropologist, is also engaged in consumer research for the California Energy Commission and for automaker BMW, which has leased about 500 experimental Mini Coopers that have been converted to all-electric propulsion and have a 100-mile-per-charge range. Turrentine is studying how drivers use and respond to the car and has already seen some surprising results. Drivers, for instance, don't seem to worry about charging the car as much as he'd expected. They'll go several days without plugging in, rather than obsessively recharging every night. He says his findings suggest that generating consumer acceptance of electric cars may be easier than most carmakers seem to believe.
"They really love these cars," he says. "And these drivers have plenty of other premium vehicles to choose from. Yet they choose to drive the EV."