Could Geoengineering Help Save the World’s Ice?
Some researchers hope so. Here are their proposals.
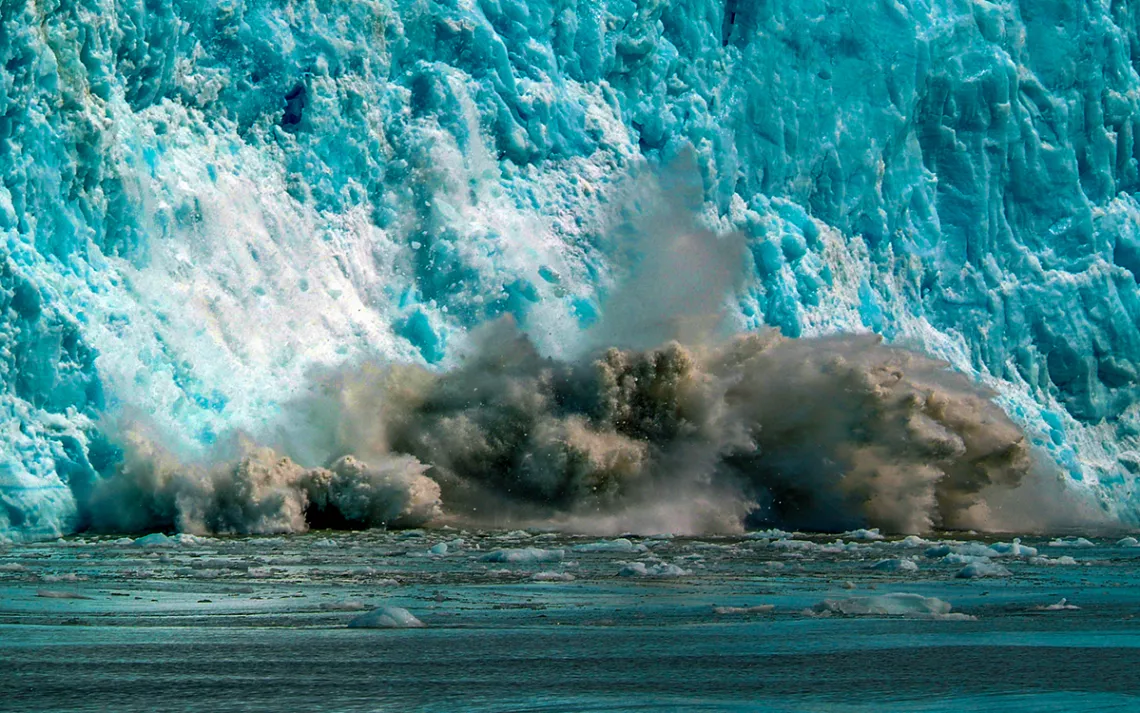
Photo by Mantaroski/iStock
Imagine 2,000 elephants running into the ocean.
The Greenland ice sheet loses that much water in weight every second, according to Dartmouth climatologist Erich Obsterberg. It equals about 250 billion tons per year. Arctic sea ice as a whole has gone from 7 million square miles in 1979 to a little over 4 million in 2019. Meanwhile, Antarctica is wasting away at six times the rate it was 40 years ago.
Thanks to climate change, we are saying goodbye to ice. Some of us, literally, are having funerals. Hundreds gathered in the Swiss Alps last September to bid farewell to the Pizol Glacier, which was once a flowing river of ice but is now a static chunk of snow. As our glaciers and ice sheets shrink, we lose freshwater resources, and without its icy reflective blanket, our planet absorbs more heat from the sun, accelerating climate change.
What we lose in land ice, of course, is also gained in sea level. Even if we manage to limit warming to 2 degrees Celsius, the world will experience greater than eight inches of sea level rise by 2100, according to the Intergovernmental Panel on Climate Change. The instability of West Antarctica's Thwaites Glacier—roughly the size of Florida—is of particular concern to scientists. Slowing the rate of ice melt there and elsewhere would allow for critical adaptation in at-risk areas, such as islands and coastal cities. Some researchers are looking to geoengineering to accomplish this.
Michael Wolovick, a researcher at Beijing Normal University, is one of them. He and his colleague John Moore have studied a number of geoengineering strategies to prevent or slow the collapse of Thwaites Glacier. “The animating idea I had was trying to find high leverage, where you have the most societal impact for the smallest-scale intervention,” Wolovick says.
Of course, the best way to prevent the collapse of ice sheets is to limit warming. Scenarios are far less severe even at just 1.5 degrees Celsius. “Geoengineering is not any substitute for emissions reduction,” Wolovick cautions.
Nevertheless, for scientists like Wolovick, the idea of tinkering more, rather than less, with our environment offers hope that we can prevent, or at least forestall, some of the worst effects of climate change. Here are some of the ideas that they are exploring for saving the planet’s ice.
A massive underground wall or "sill." | Illustration courtesy of Michael Wolovick
Underwater Sills
One proposal is to build a massive underground wall or “sill” at the ice-ocean interface of key glaciers like Thwaites.
This interface is where warm water eats away at the glacier, elongating the shelf that floats above the ocean. Like slowly hacking away at the base of a tree, the rest of the system becomes wobbly. The shelf increasingly calves into icebergs and melt accelerates.
A barrier, like an earthen dam built from ocean floor material, could block that warm water. It could also provide a physical “buttress” on which a glacier might stabilize and thicken. Wolovick’s models of Thwaites show that if the most extreme, largest sill were built to block the warm water completely, the success rate would be 100 percent, while less extreme options show success rates between 30 and 90 percent.
But an engineering feat of this scale would be enormous, according to National Snow and Ice Data Center scientist Twila Moon.
“The idea of building such a big piece of infrastructure would require a logistical effort that I think might not even be possible. . . . That’s an area that even research ships have trouble getting to on a year-to-year basis,” Moon says. Plus, she added, “Ice sheets have been pretty good at plowing through obstacles of all sorts as long as they’ve existed.”
All of Wolovick’s models begin 100 years in the future, leaving time, he thinks, for us to hash out the mechanics and test on smaller glaciers first. “I don’t expect to be alive when humanity tries to control Thwaites Glacier,” Wolovick says. “I’m a young guy but . . . it’s important to start planning.”
Drying the glacial bed
Another idea Wolovick and Moore suggest is drying out a glacier’s bed—the bottom of the glacier where ice meets rock and meltwater flows in between the two. That meltwater lubricates the ice, like adding soap to a slip-and-slide, accelerating glacial movement and mass loss.
If we drilled a tunnel to the bed and pumped water from beneath to the surface, we could hypothetically refreeze the ice-rock interface and slow glacial movement. The feasibility of drilling thousands of feet through ice and properly draining tonnages of water is fuzzy, but it has been done on a small scale. In Engabreen, Norway, meltwater at the base of the Svartisen’s glacier is pumped to a hydropower plant.
Mass deposition
One way to stop ice loss is to create a whole lot more ice. Johannes Feldman and his colleagues from the Potsdam Institute suggest artificially thickening glaciers by dumping sea water back onto ice sheets for it to refreeze.
Last July, they published a paper in Science Advances showing that mass deposition could stabilize West Antarctica under certain conditions. However, they write, “The practical realization of elevating and distributing the ocean water would mean an unprecedented effort for humankind in one of the harshest environments of the planet.”
You would need a “hell of a lot of snow,” says Wolovick.
Pumping that water (over an area equivalent to the size of Costa Rica, in their model) would be extremely energy intensive. The authors proposed using 12,000 high-end wind turbines to power the process. Indeed, Antarctica is rather windy, but even if this idea were doable, Wolovick doesn’t think it’d have any lasting impact.
“Over the course of a few centuries, the ice will just drain that extra mass into the ocean,” he says.
Leslie Field in the Arctic | Photo courtesy of Ice911
Silica Beads
Leslie Field wants to enhance the Arctic’s reflectivity—with beads.
Her company, Ice911, is testing a technology to spread silica beads on snow and ice to spur sea ice growth. While sea ice does not impact sea level rise (think about a glass of ice water—when the ice melts, the volume of the water is still the same), it does decrease how much heat the Arctic absorbs from the sun because ice reflects more energy than open ocean.
A chemical engineer and lecturer at Stanford, Field was first inspired to act against climate change after seeing An Inconvenient Truth. She began wondering what a person like herself could do, and as a material scientist, asked herself, “What safe material could you use to replace the loss of reflectivity in the Arctic?”
After years of testing in water-filled blue buckets on her porch in California, she settled on silica, a naturally occurring compound in all ecosystems, with high reflectivity.
The technology is in ecotoxicology and field testing. So far, it has shown positive results and no adverse ecological effects. If Ice911 proves safe and effective, Field hopes to hand off the technology to a collaborative body like the UN and the Arctic nations in three to five years. “I know very well that people can fall in love with their own ideas,” she says, “so you need outside regulation.”
But is geoengineering really a good idea?
Practical problems aside, many environmentalists object to geoengineering on principle, seeing it as nothing more than an excuse to let fossil fuel companies continue business as usual. (“It’s an engineering problem, and it has geoengineering solutions,” then–ExxonMobil CEO Rex Tillerson said about climate change in 2012.)
Field, herself a former employee at Chevron, would rather think of Ice911 as a strategy for “climate restoration”—the act of repairing something we have harmed.
Ideas for geoengineering also vary widely, from spraying aerosols—a highly controversial proposal—to carbon capture technology, which most scientists think will be a necessary part of limiting warming.
Given that range of “methods and implications,” Moon says, “you can’t really say geoengineering is good or bad.”
What we can say is that geoengineering is very complicated. The political, social, ecological, economic and moral consequences of Wolovick and others’ proposals are far from fleshed out. How would a giant underwater wall impact wildlife? Who would pay for these projects? What governing body would oversee the process, like in Antarctica, a continent ruled by international treaties? What about the Indigenous communities in the Arctic—do they get a say? What if these technologies give humanity a license to ignore emissions because we have an “easy fix”?
Ultimately, Moon thinks our resources are better spent adapting and mitigating in the present.
For Wolovick, geoengineering offers a contingency plan for the future.
But “in the long run, the fate of ice sheets is closely tied to cumulative CO2 emissions,” he says. “If we keep emitting carbon without bound, the only reasonable goal of glacial geoengineering would be to slow the rate of collapse.”